“Transposons are mobile genetic sequences that change their position within the genome through a process called transposition, which generates a new copy of the gene at a different location.”
Our genome has many mysteries inside, like our universe. As a large part of the genome is non-coding, the very tiny portion governs nearly all the biological activities in us. The 97% part of our genome is non-coding.
This non-coding, ‘dark matter’ contains various types of repetitive elements. Transposons are one such element of our genome. It is a non-coding, intermediately repeated and inactive genomic island that could move between two genomic locations.
Sounds exciting!
Let’s understand the transposons and transposition mechanism along with various types of TEs in this article.
Disclaimer: The content presented herein has been compiled from reputable, peer-reviewed sources and is presented in an easy-to-understand manner for better comprehension. A comprehensive list of sources is provided after the article for reference.
Abbreviation used in this article
Abbreviation | Full name |
DNA | Deoxyribonucleic acid |
LINEs | Long Interspersed Elements |
SINEs | Short Interspersed Elements |
LTR | Long Terminal Repeats |
UTR | Untranslated Region |
MIR | Mammalian wide-interspersed Repeats |
TE | Transposable elements |
TSD | Target site duplication |
TIR | Terminal Inverted Repeats |
MITE | Miniature Inverted Repeats |
mRNA | Mature RNA |
Key Topics:
Introduction
DNA can not move!
Genes have a fixed location on chromosomes. The overall structure of our genome is invariant. The whole transcriptome is responsible for various types of phenotypes and traits in an organism.
The discovery of the transposable genetic elements challenged the fact that DNA can not move. The transposons are also called transposable elements which are mobile genetic sequences that can move from one place to another into the genome.
That means, it can change its location, research suggests. Both prokaryotes and eukaryotes possess TEs in their genome. In fact, they are active in bacteria and other prokaryotes.
This “selfish” and “parasitic entity” can produce mutations, inactivate genes and produce genomic instabilities. It is also responsible for chromosomal breakage as well. These elements are also known as jumping genes.
Related article: Role of Microsatellite Instability (MSI) in cancer (MSI-H, MSI-I and MSS)
History of the transposons:
Between 1944 and 1945, Barbara McClintock discovered the first transposons in the maize through genetic instability. She called it transposable elements.
She noticed that the chromosomal breakage occurred due to the presence of the mobile element at the site of the breakage.
For her discovery, B. McClintock was awarded the Nobel Prize in the year 1983 for Physiology and Medicine.
She named it as Ac-Ds -types of transposable elements.
Her discovery is an important milestone in genetic science so it is essential to understand what she observed or discovered during her experiments.
Let’s understand her experiments,
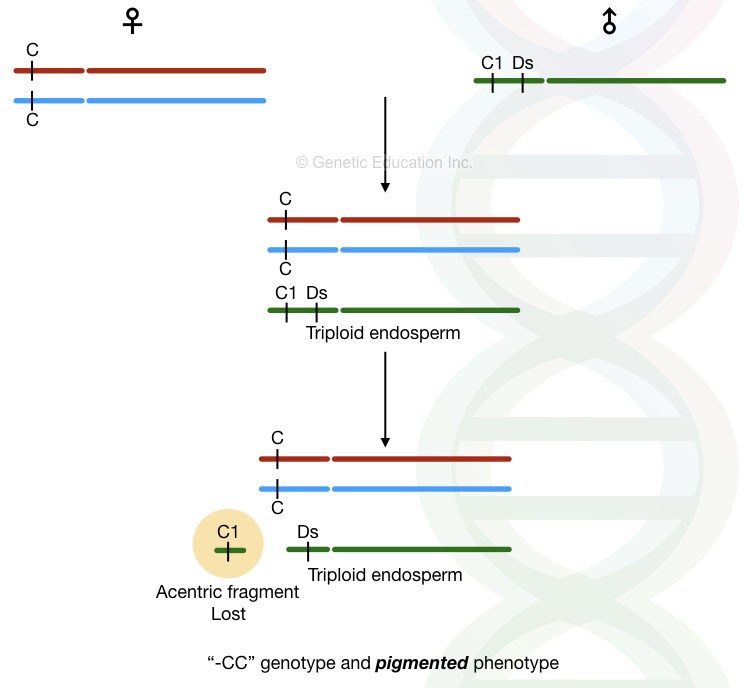
The triploid endosperm of the Maize comprises two maternal and one paternal nucleus. See the above figure.
The outermost layer of the endosperm is called an aleuron. Transposons causes aleuron pigmentation. She selected a marker on a short arm of chromosome 9 and named it C. The CC genotype is responsible for the pigmentation of the aleurons.
On the contrary, C1 is the dominant inhibitor of the coloration on the endosperm. She crossed both types of plant and observed C1CC genotype in the F1 progeny. Theoretically, the F1 progeny must contain colorless kernels. Although the F1 progeny contains colorless kernels not all.
Some of the kernels show a brownish-purple color on the outer layer of the endosperm. In the presence of the C1 dominant inhibitor, none of the progenies should have the coloration which means the C1 inhibitor gene is lost. She concluded that during endosperm development, the C1 gene is lost due to genetic instability which produces such tissues that produce colored patches.
She named the genotype as “-CC” and postulated that the loss of the gene fragment occurred due to the chromosomal breakage.
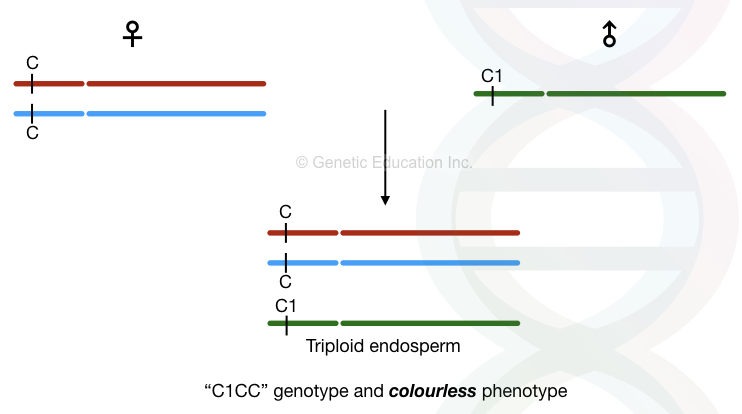
She experimented several times and concluded that some factors present on chromosome 9 are responsible for the chromosomal breakage, she named it “Ds elements” or dissociation factor.
However, she noticed that these elements cannot be activated without a special class of activators. The activators help the Ds elements to induce the chromosomal breakage and lead to a transposition of some of the genes.
She named it “Ac elements” or “Activator“.
This class of transposons is called Ac-Ds transposable elements. She noticed that apart from the Ac-Ds transposons there are other different transposable elements present in the maize genome.
~90% of the maize genome is made up of mobile jumping transposable elements. Further, 44% of the human genome is made up of transposable elements.
“The Ac elements are functionally autonomous, self-activated whereas the Ds elements are functionally non-autonomous, requiring activators to do their function.”
After McClintok, the story of mobile genetic elements has gained popularity in the genetic fraternity. Let’s see what exactly these mobile genetic elements/ transposable elements or transposons are!
What are transposons?
As we discussed earlier, the transposons are the mobile genetic elements.
Recombination is the main event transposons can move within the genome, at nearly any place- randomly while replication is the main culprit that helps them to spread. Theoretically, it’s an insertional mutation.
Interestingly, future studies suggested that transposons can even move between two different genomes. For instance, retrotransposons— retrovirus-derived transposons can be incorporated into the host genome and move in there!
One hypothesis also suggests that viruses, in this way, are derived or evolved from transposons.
Some of the characteristics of the transposons are enlisted below,
- They are mobile genetic elements that can move from one place to another into the genome and between the genomes.
- Transposons are intermediately repeated sequences.
- Transposons are present in either active or inactive form.
- They can be either replicative, where they create a new copy through replication, or non-replicative, where they move to another location without replicating.
- Transposons are ubiquitous.
- It can jump through both DNA and RNA intermediates.
- It does not affect the original function of a gene, directly.
- It can interrupt gene expression.
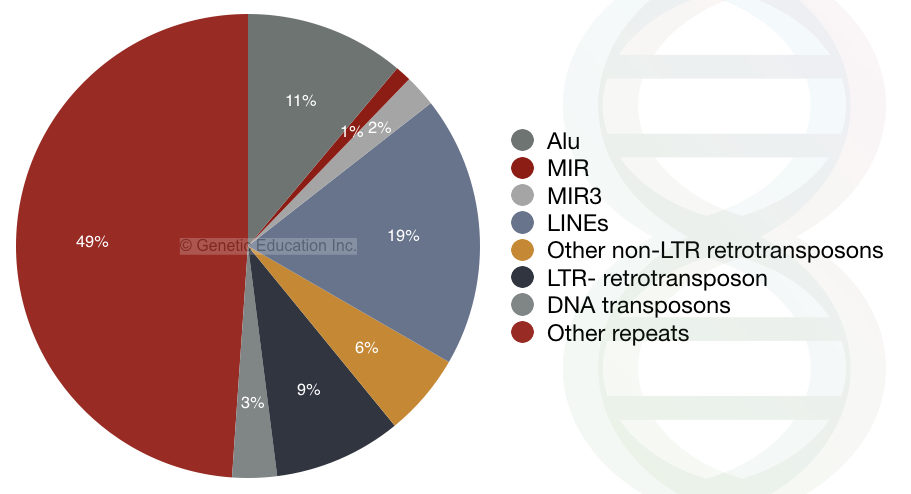
Transposons in some of the species:
Specie | % of transposons |
Human genome | ~45% |
Mouse genome | 37% |
Fish | 10% |
C. elegans | 12% |
Maize | >80% |
Now we need to understand how transposons affect a gene’s function!
As aforementioned, recombination-mediated insertion or deletion are two ways transposons affect a gene’s function. The transposition mechanism needs more elaborative and in-depth understanding; for this article, I am giving you just a brief overview.
For instance, if the transposon is integrated into the coding gene region, it can affect the gene’s function partially or fully. If a gene segment is present between two correctly oriented transposons then that segment moves along with them and causes deletion. This occurs due to target site duplication (click the link and read the article for more details).
In both conditions, a gene’s normal function or expression can be altered partially or fully. Now you know why these fascinating mobile genetic elements are parasitic to the host!
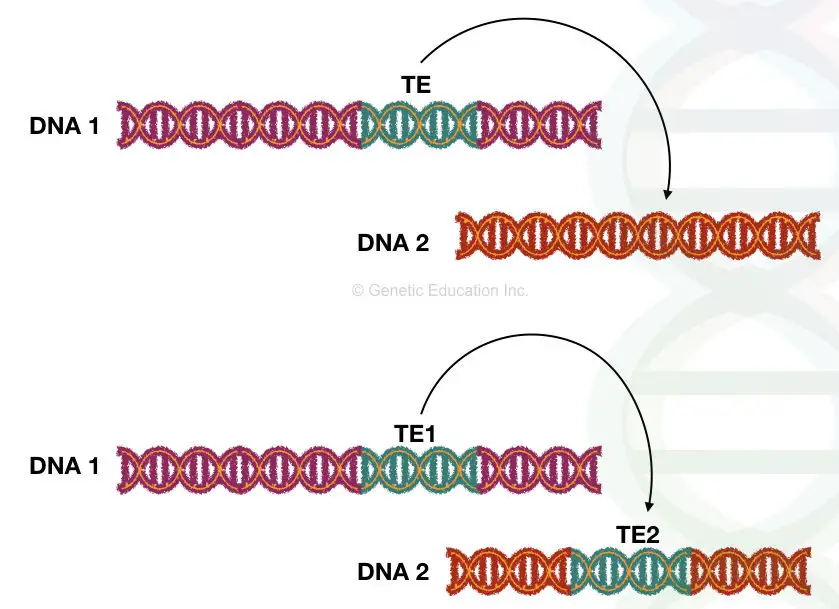
Different types of transposons:
The type and number of transposable elements vary among various organisms. Go through the table first. This section majorly focuses on the various types of transposons. We will explain transposons in eukaryotes and prokaryotes.
Let’s begin with a broad classification. Two broad classes of transposons are:
- DNA transposons
- Retrotransposons
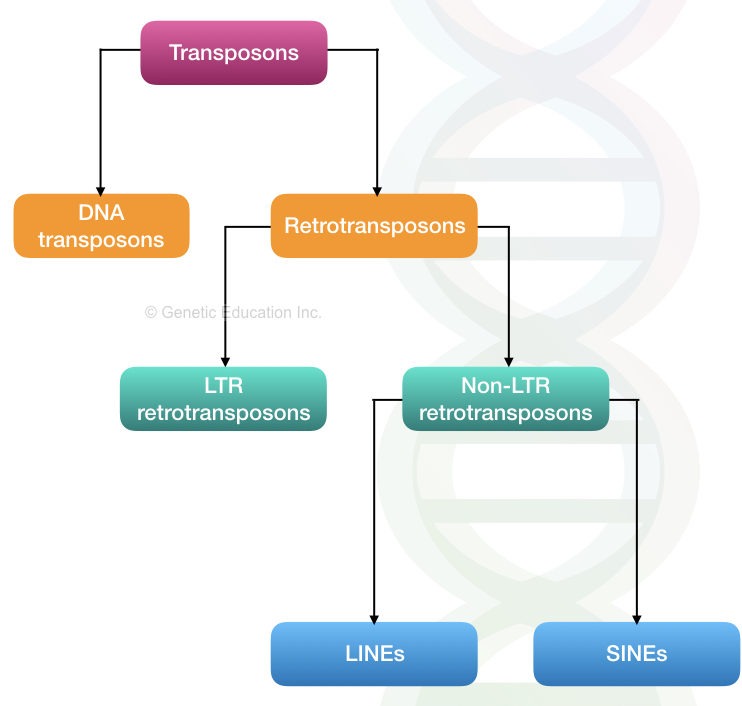
Retrotransposons:
Retrotransposons occupy the larger portion of the eukaryotic genome.
They are continuously replicated by the “copy and paste” mechanism. The reason why retrotransposons possess a large portion of the eukaryotic genome is their continuous replicating nature.
Reverse transcription much like the retrotransposons! Is the main mechanism for retrotransposition. Let me explain how.
First, it transposes to the active gene location and transcribes into the mRNA. Although it jumps through this RNA intermediate, it can not be integrated into the genome. By taking the help of the reverse transcriptase enzyme the RNA is converted into the cDNA.
Now, it can be integrated into another location. Read this article to learn this sub-topic in detail: Replicative transposition.
Structurally, transposons contain repeats at their ends. Half of the retrotransposon contains long terminal repeats (LTRs). Approximately 1000 bp long terminal repeats are present on both ends of the retrotransposon. Note that a general transposon structure is explained in another article, you can read it by clicking the link.

The above structure of retrotransposons showed two genes— “gag” and “pol” that encode protein integrase, RNase H, reverse transcriptase and help in autonomous transposition. Interestingly, retrovirus contains both these genes and a dedicated gene for the viral envelope.
That “env” gene in retrovirus makes them unique. Due to this, scientists also hypothesized that the retrovirus originated from the retrotransposons. Retrotransposition makes a gene inactive.
Studies showed that >50% of the human genome consists of retrotransposons. Nonetheless, they are endogenous and inactive now.
The rest of the retrotransposon does not contain LTRs and so-called non-LTRs which are further categorized into LINEs and SINEs.
LINEs:
The LINEs are ‘Long Interspersed Elements’ found in the higher eukaryotes. These are 6 to 8 Kb long interspersed repeat retrotransposons. Structurally, it contains two open reading frames, ORF1 near the 3′ end and ORF2 adjacent to this. See the figure below,

It also contains the promoter region for the RNA polymerase (because it is involved in reverse transcription). The ORF1 encodes for the reverse transcriptase whereas the ORF2 encodes for the endonuclease.
The reverse transcriptase synthesizes DNA from the mRNA template. Finally, a process called retrotransposition inserts into another genomic location. The 5’ end of the LINEs is truncated as replication initiates from the AT-rich 3’ end of the retrotransposon.
The LINEs subfamily, LINEs1, LINEs2, LINEs3 and LINEs4 account for ~20% of the human genome in which the LINEs1 contains 500,000 copies (~17%) of the total sequences.
SINEs:
SINEs are the main identity of the eukaryotic metazoan. The metazoa contains large numbers of the non-autonomous transposons called SINEs. The SINEs are shorter transposons of 300 to 400bp having RNA polymerase III promoter region.
The sequences of the SINEs are similar to the (3′ end) sequences recognized by reverse transcriptase. Most SINE elements are non-autonomous.
When we are talking particularly about the human genome, it contains three families of the SINE elements.
- Alu
- MIR
- MIR3
Most copies of the Alu transposons contain restriction sites for the Alu1 restriction endonuclease. Hence the name is derived from there. Interestingly, 10% of the genome is made up of the Alu type of SINEs transposable elements. It is the most common type of transposon with approximately 106 copies present in our genome.
On average after every 3Kb of genomic portion, one Alu element is present. Notedly, Alu are actively present in our genome but are not uniformly distributed. Instead of the tRNA gene, the Alu elements are derived from the 7SL RNA.
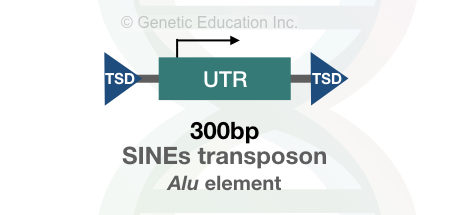
The MIR and MIR3 are different.
MIR- mammalian wide-interspersed elements are found in all mammals. MIR and MIR3 contain sequences similar to the recognition sequence of the reverse transcriptase enzyme’s 3’ end. Its sequence structure is a bit different from the Alu elements.
The reverse transcriptase (encoded by the LINEs 2) recognizes the 50 bp sequence present on the 3′ end of the MIR3, similar to its recognition site. LINEs and MIR elements are inactive in the genome now. The last active LINE 3 elements disappeared 100 million years ago.
13% of the human genome is made up of Alu, MIR, and MIR3 transposons.
LTR- retrotransposons:
The long terminal repeat retrotransposons are very much similar to the retrovirus. It contains the gag and pol genes for the synthesis of integrase, RNase H and reverse-transcriptase. Due to the presence of the “gag” and “pol”, the LTR-retrotransposons follow autonomous transposition. Interestingly, the LTRs are more similar to the retrovirus. The retrovirus contains only an additional “env” gene for the coat protein.

The LTR-derived retrotransposons contain ~8% portion of the genome. These transposons have been inactive now.
DNA transposons:
Bacteria and some other prokaryotes possess active transposons referred to as DNA transposons. Studies suggest that these transposons give bacteria antibiotic resistance power. To know more read this article: Role of Transposons in Bacterial Antibiotic Resistance.
Through the copy-and-paste mechanism, transposition leaves a copy behind. This will generate various copies of an antibiotic resistance gene and help bacteria survive against antibiotics.
Interestingly, DNA transposons spread multidrug resistance effectively. Structurally, between the end terminal repeat, DNA transposons contain a ‘transposase’ or ‘transponase’ encoding gene. Transposase mediates the DNA transposition process.
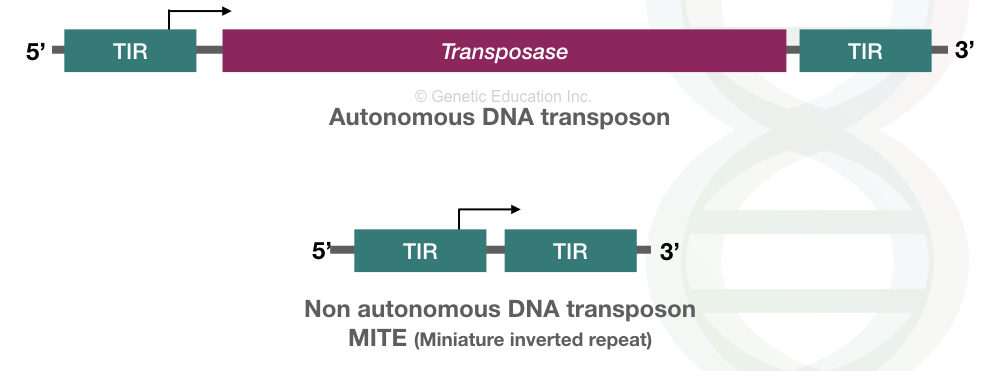
Nonetheless, in the human genome, the DNA transposons have been inactive before 50 million years ago.
In eukaryotes, particularly in humans, only ~3% of the whole genome comprises DNA transposons, however, they were inactive before 50 million years ago.
Moving ahead.
DNA transposons are further categorized into class II DNA transposons. Let’s take a look at the differences between class I and class II transposons.
Feature | Class I Transposons (Retrotransposons) | Class II Transposons (DNA Transposons) |
Enzyme Required | Reverse transcriptase | Transposase |
Transposition Mechanism | Requires reverse transcription (from RNA to DNA) | Direct transposition (from DNA to DNA) |
Presence in Viruses | Actively found in viruses | Not typically associated with viruses |
Presence in Eukaryotic Genome | Inactively present | Inactively present |
Presence in Prokaryotic Genome | Not commonly found | Actively present, especially in bacteria |
Capability of Transporting Genes | Typically does not transport additional genes | Can transport additional genes |
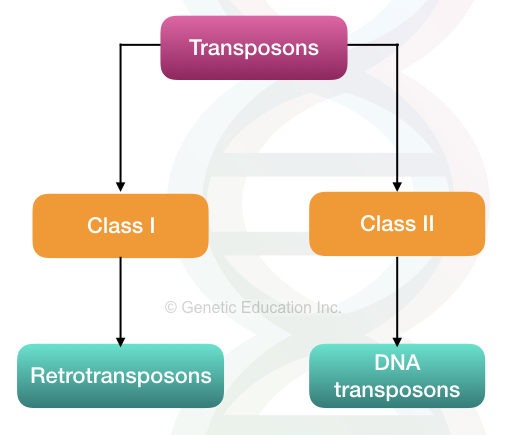
Conclusion:
In conclusion, transposons are an integral part of our genome, but are ‘mostly’ inactive, because we no longer need them. It. However, they played a crucial role in the evolution process to produce new genotype combinations.
Bacteria still have active transposon copies that help me to develop resistance against drugs. Largely, TEs became inactive 10 million years ago, at least in eukaryotes. Scientists are still struggling to understand transposons, their main agenda and why they become dormant.
Interestingly, active bacterial transposons are used in site-directed mutagenesis and gene transfer techniques. We will cover an entire article on this topic.
I hope you like this article. Do share it and subscribe to our blog.
Resources:
Bourque, G., Burns, K.H., Gehring, M. et al. Ten things you should know about transposable elements. Genome Biol 19, 199 (2018). https://doi.org/10.1186/s13059-018-1577-z.
Muñoz-López M, García-Pérez JL. DNA transposons: nature and applications in genomics. Curr Genomics. 2010 Apr;11(2):115-28. doi: 10.2174/138920210790886871. PMID: 20885819; PMCID: PMC2874221.
Subscribe to our weekly newsletter for the latest blogs, articles and updates, and never miss the latest product or an exclusive offer.